By Emma Hoffman
Before conducting research on this series of three objects, the idea of a radiation detector was so foreign to me that it almost seemed like an imaginary instrument created for science fiction movies like “Blade Runner” or “The Matrix.” I was intrigued by the radiation detector’s box that read: “nuclear-chicago Radiation Detector”; and decided, not knowing what it contained, to pluck the mysterious container off a shelf in UTSIC’s storage facility. Alongside the radiation detector, the instruments in this series include a clear plastic cylinder containing a stack of what look like thin, circular, aluminum poker chips, as well as a light-grey, cube-shaped timer. What were these instruments used for, and why was someone trying to detect radiation at the University of Toronto? I was curious to find out more about the instruments.
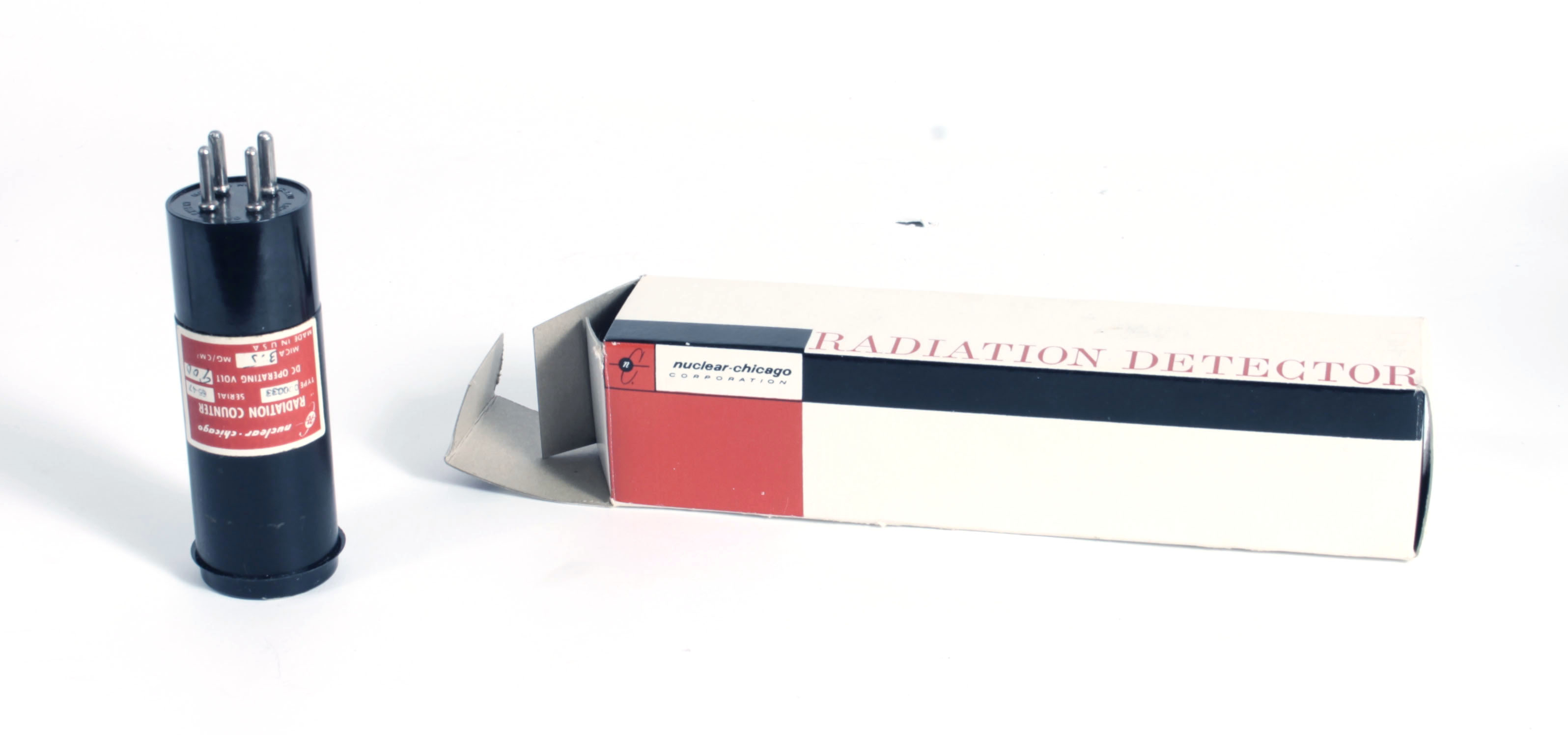
Pre-cursors to Contemporary Radiation Research
My first step was to engage in research about radiation. Radiation occurs when an unstable atom decays, consequently releasing radioactive energy. It was first discovered in 1896 when scientist Henri Becquerel was experimenting with developing photographic plates by wrapping them around minerals and exposing them to the Sun (McMahan, 2002). One cloudy day, Becquerel put the mineral sample in his desk drawer, and discovered that the photographic plate had still been exposed without sunlight (Ibid.). Thus, Becquerel realized that this occurred not because of the Sun’s affects on the plate as he had originally hypothesized but because of natural radioactivity from the mineral that had the ability to develop the plate inside the drawer (Ibid.). Although Becquerel had discovered the effects of radiation during the late-19th century, it was not until the late-1920s that radiation laboratories became part of scientific practice in universities. Contemporary research using radiation detection originated from scientist Ernest Orlando Lawrence’s invention of the cyclotron instrument, which he began to create in 1929 to solve the problem of having to use long tubes for radiation detection (American Institute of Physics). His research at University of California, Berkeley was also crucial toward developing North American physics programs at universities, and toward the expansion of research labs and research teams, which—converse to pre-World War Two labs which usually consisted of a scientist and a couple assistants—now required hiring larger numbers of educated scientists, engineers, multi-disciplinary scholars, and assistants to run larger-scale research projects and experiments (Ibid.).
Nuclear-Chicago
Upon looking up: “nuclear-chicago,” I discovered it was a company that manufactured instruments for use in radiation detection. The company was originally founded in 1946, in Chicago, Illinois as Instrument Development Laboratories, Inc. by James Schoke, an American nuclear physicist who is best known for his involvement in the Manhattan Project: the U.S. government’s research project that worked to produce nuclear weaponry during the Second World War, and the initiative that produced the first atomic bomb. The Manhattan project (named for the location of most of the earlier research for the project) was crucial to the development of radiation detection instruments. During World War Two, information on the measurement of radiation was considered classified government property and was given code names, like “Manhattan,” until after the late-1940’s when most of this information on radiation testing became available to the public (National Radiation Instrument Catalog, 2014). Interestingly enough, physicists working on the Manhattan Project contacted Disney for the rights to reference Disney characters in their code names for their scientific instruments: such as a radiation meter named “Pluto.” However, the incognito code names and secretive monikers for scientific instruments were dropped after the War, and Instrument Development Laboratories continued to manufacture instruments and chemicals for medical research and became a public enterprise (Atomic Heritage Foundation, 2016). It was actually nine years after its initial establishment, in 1954, that the company changed its name to nuclear-chicago (National Radiation Instrument Catalog, 2014).
What are these instruments?
I discovered the scientific names and functions for these instruments while browsing through historic nuclear-chicago catalogs and found similar objects to the three instruments in the series in nuclear-chicago’s “Catalog Q,” which was published in 1957. I knew this catalog was dated slightly earlier than the provenance of the instruments because the address printed on the last page of the catalog was 223 West Erie Street, Chicago, Illinois. This address differed from the address on the box containing the radiation detector which was marked: “333 EAST HOWARD AVENUE DESPLAINES, ILLINOIS,” and I learned that this was because nuclear-chicago moved its facilities from Chicago, to Des Plaines in 1959 (Ibid.). Considering this information, the date of the radiation detector had to be after 1959, and thus, the catalog was not completely accurate for the purposes of my provenance research. However, I was thankfully able to find images of all three components of the instrument in the catalog, which helped with classifying and naming the instruments.
The technical name for the radiation detector is a “D34 Geiger-Muller thin-window radiation detector.” The Geiger-Muller detector is the central part of a Geiger counter: an instrument that scientists began using to measure, count, and detect radiation during the late-1940’s and 1950’s. The Geiger counter was designed to detect radioactive isotopic rays including Gamma rays, beta rays, alpha rays, and x-rays (“About Geigers”). Typically, Geiger counters are used to test for radioactivity in the environment near a nuclear radiation facility, to check levels of radiation in cancer patients, to check for radiation leaks in medical facilities, and even to assess potential radiation levels of archaeologically excavated materials (“Applications”).
Dr. Gordon M. Clark’s Radiation Lab and Radiation Detection at UofT
These instruments were used by Professor Gordon M. Clark who was a leading expert on the effects of radiation, and conducted research in a radiation facility in the basement of Ramsey-Wright Laboratory at University of Toronto. The laboratory, which was part of the former Zoology Department at UofT, was built during the building’s initial construction in 1963, and was built, at the request of Professor Clark, specifically for use as a radiation laboratory, using proper concrete and lead insulation for safe radiation procedures. The laboratory was situated in Ramsey-Wright so that radiation in the lab would be directed away from the building, and the seemingly innocuous grass-covered mound—that now has an iron sculpture of a moose on top of it at the southwest corner of Harbord and St. George—was actually commissioned by Dr. Clark to absorb any radiation being emitted from the facility.
As detailed by one of Professor Clark’s former students, Dr. Clark’s research was focused on the effects of low-dose radiation on mammalian systems and he used radiation detection instruments in his own work, and for student’s experiments. Dr. Clark was the official supervisor for all experiments using radioactive materials at UofT and helped to train both students and faculty on safe usage of radioactive isotopes. Dr. Clark mainly experimented on mice and rats and produced research on the response of mice to radiation exposure. However, he also collaborated with Dr. Chandorkar: a Botany professor, to study the effects of radiation on plant systems. Furthermore, Dr. Clark experimented with radiation on the growth of seedling pine trees in order to determine whether nuclear power plants had dangerous effects on the environment.
Dr. Clark used the radiation detector alongside a 250kVp X-ray machine to measure radiation in plants and mammals. The x-ray unit was used to deliver high doses of radiation to both living and dead specimens, and the radiation detector was used to measure the response of ions to the radiation. The thin-window, or mica sheet, on the end of the detector, is a feature that was designed to detect lower-energy particles (like beta and alpha rays), and to be able to determine what type of radiation was being emitted from a specimen.
The cylindrical container accommodating the poker-chip-like aluminum circles is actually a container of counting planchets that were used to weigh biological samples that had been exposed to radiation. Dr. Clark used the counting planchets in his experiments to weigh excised tissue and organs from rats and mice. The planchets were typically used to weigh tissues from the mammals’ spleens and thymus’ that were used during experiments. Tissue from these organs would be weighed twice using the planchets: once right after the tissue was extracted from the animal, and again once the tissue was dried out, most likely to preserve the samples for future
examination.
The cube-shaped timer, a T1 dual timer, was used with a scaler unit: a machine that counts the effects of radiation on a sample, over a specific time period. The timer would be set to begin counting at a predetermined time and this would also trigger the scaler unit to begin counting radioisotopes. When the timer was finished counting down, the scaler would also stop and would provide the researcher with a measurement of radioisotopes based on the amount of particles per. time value.
Implications of Radiation Research at U of T
As U of T’s Zoology Department was discontinued about ten years ago, it was difficult to find traces of the radiation research that once existed in the basement of Ramsey-Wright. However, I was lucky to be able to visit the former radiation facility that is now a storage area for the Department of Ecology and Evolutionary Biology at U of T. One of the only traces of material culture that remains of Dr. Clark’s former laboratory is a circular viewing portal that looks from what is now one storage room into the next. Similar in structure to a porthole in a submarine, the viewing portal was used in Dr. Clark’s radiation lab to view radiation experiments on the other side of a concrete-insulated wall, sealed by two airtight doors. Although the laboratory in which he experimented no longer exists, Dr. Clark’s legacy at U of T still lives on through the laboratory that he helped to create and supervise, and through his promotion of research into radiation medicine and environmental radiation—research that set a precedent for the boundless scientific discovery that continues today at the University.
This post is dedicated to the late Dr. Gordon M. Clark, WWII veteran and Professor, who passed away almost a year ago, on May 5, 2015, at the age of 90.
References
American Institute of Physics. “The First Cyclotrons.” (2016). AIP. Retrieved from https://www.aip.org/history/exhibits/lawrence/first.htm.
Atomic Heritage Foundation. (2016). “James Schoke.” Atomic Heritage Foundation. Retrieved from http://www.atomicheritage.org/profile/james-schoke.
geigercounters.com. (n.d.). “About Geigers.” Geigercounters.com. Retrieved from http://www.geigercounters.com/AboutGgr.htm.
geigercounters.com. (n.d.). “Applications.” Geigercounters.com. Retrieved from http://www.geigercounters.com/GigrApps.htm.
McMahan, Peggy. (2002, February). The ABC’s of Nuclear Science. Lawrence Berkeley Laboratory, Nuclear Science Division. University of California, Berkeley. Retrieved from https://www.nuc.berkeley.edu/sites/default/files/events/teachers-workshop/abc.pdf.
National Radiation Instrument Catalog. (2014). “nuclear-chicago.” National Radiation Instrument Catalog. Retrieved from http://national-radiation-instrument-catalog.com/new_page_42.htm.
Nuclear-Chicago. (1957). “Catalog Q.” nuclear-chicago corporation. Retrieved from http://www.orau.org/ptp/Library/Catalogs/Nuclear%20Chicago%20Catalog%20Q%20small.pdf.